The RNA Mobility Shift Assay, often referred to as RNA EMSA (Electrophoretic Mobility Shift Assay), is a biochemical technique used to study the interaction between RNA molecules and proteins or other molecules. It is similar to the DNA EMSA, which is used to study DNA-protein interactions. RNA EMSA is a versatile tool in molecular biology and biochemistry research and has several applications, including the investigation of RNA-protein interactions, RNA secondary structure probing, and the study of RNA-ligand interactions.
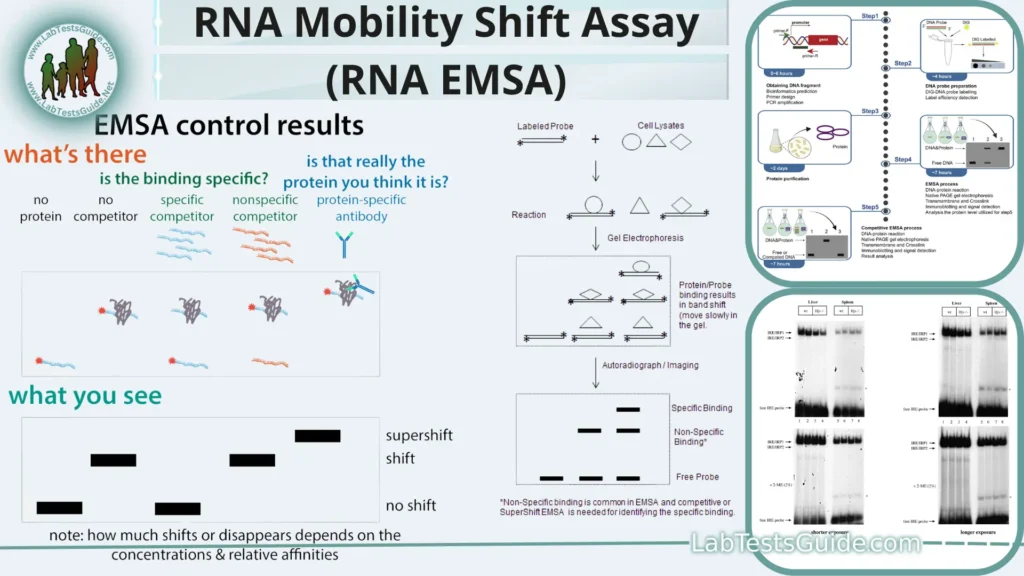
- Principle: RNA EMSA uses electrophoresis to study RNA-protein or RNA-ligand interactions by separating complexes from free RNA.
- Components: Requires labeled RNA, proteins/ligands, and specific buffers.
- Procedure: Mix RNA and proteins/ligands, run on a gel, and visualize shifts.
- Interpretation: Shifted bands indicate complex formation; free RNA migrates faster.
- Applications: Used to study RNA-protein interactions and ligand binding.
- Advantages: Provides insights into binding specificity and affinity.
- Limitations: Sensitivity to experimental conditions and potential artifacts.
- Optimization: Troubleshooting tips for better results.
- Emerging Technologies: Evolving methods in RNA interaction studies.
- Significance: Contributes to understanding RNA biology and drug development.
- Future Directions: Potential advancements in RNA EMSA and its expanding role in research and industry.
Abstract:
RNA Mobility Shift Assay (RNA EMSA), a vital technique in molecular biology and biochemistry, plays a pivotal role in deciphering RNA-protein and RNA-ligand interactions. This article provides a comprehensive overview of RNA EMSA, delving into its fundamental principles, experimental procedures, interpretation, and applications. RNA EMSA is a powerful tool for investigating the intricacies of RNA biology, offering insights into binding specificity and affinity, with wide-ranging applications in research and drug discovery. While highlighting its advantages, limitations, and optimization strategies, we also explore emerging technologies and future directions in this field, emphasizing its indispensable contribution to advancing our understanding of RNA-related processes.
Principles of RNA EMSA:
- Electrophoresis: RNA EMSA relies on electrophoresis, a technique that separates molecules based on their size and charge when subjected to an electric field.
- Complex Formation: RNA molecules can form complexes with proteins or ligands, which alter their mobility in an electrophoretic gel.
- Gel Matrix: A gel matrix, typically polyacrylamide, retards the movement of larger RNA-protein or RNA-ligand complexes, causing them to migrate more slowly than free RNA.
- Shifted Bands: The appearance of shifted bands on the gel indicates the presence of RNA-protein or RNA-ligand complexes.
- Free RNA: Unbound or free RNA migrates faster through the gel, resulting in distinct bands.
- Binding Specificity: RNA EMSA helps assess the specificity of RNA-protein or RNA-ligand interactions.
- Binding Affinity: The intensity of shifted bands provides insights into the binding affinity between RNA and interacting molecules.
- Detection: Shifted bands are visualized using techniques like autoradiography for radioactive labels or fluorescence for fluorescent labels.
- Quantitative Analysis: The assay allows for quantitative analysis of binding events based on band intensity.
- Applications: RNA EMSA is widely used to study RNA-protein interactions, RNA-ligand binding, and regulatory roles in gene expression.
- Advantages: Provides a versatile and valuable tool for characterizing RNA interactions.
- Limitations: Sensitive to experimental conditions and potential artifacts, necessitating careful optimization.
Applications of RNA EMSA:
- Studying RNA-Protein Interactions: RNA EMSA is widely used to investigate interactions between RNA molecules and RNA-binding proteins. It helps identify and characterize RNA-protein complexes involved in processes like RNA splicing, translation, and gene regulation.
- Exploring RNA-Ligand Interactions: Researchers use RNA EMSA to assess the binding of small molecules, drugs, or ligands to specific RNA targets. This has applications in drug discovery, where understanding RNA-ligand interactions can aid in developing therapeutics.
- Assessing Binding Specificity: RNA EMSA allows for the determination of binding specificity, helping researchers discern which proteins or ligands interact with a particular RNA sequence. This is crucial for understanding regulatory networks in cells.
- Studying RNA Secondary Structure: By probing RNA molecules with various conditions and ligands in EMSA, researchers can gain insights into RNA secondary structure, which is important for understanding its biological function.
- Investigating Ribonucleoprotein Complexes: RNA EMSA is instrumental in the study of ribonucleoprotein complexes, such as ribosomes and spliceosomes, providing insights into their assembly and function.
- Validation of RNA Targets: In drug development and RNA-based therapies, RNA EMSA is used to validate the binding of potential therapeutic agents to specific RNA targets, ensuring their effectiveness and specificity.
- Functional Analysis of Non-Coding RNAs: Researchers employ RNA EMSA to study non-coding RNAs, like microRNAs or long non-coding RNAs, to understand their roles in gene regulation and disease processes.
- Viral RNA Research: RNA EMSA aids in examining the interactions between viral RNA and host proteins, offering insights into viral replication, pathogenesis, and potential therapeutic targets.
- RNA Engineering: In synthetic biology and RNA engineering, RNA EMSA can be used to validate the binding of designed RNA molecules to specific proteins or ligands, enabling the creation of custom RNA-based tools and devices.
- Environmental Monitoring: RNA EMSA can be adapted for environmental applications to detect specific RNA sequences in samples, such as monitoring the presence of pathogenic microorganisms in water sources.
- Structural Biology: In structural biology, RNA EMSA can complement other techniques like X-ray crystallography and NMR spectroscopy by providing information about RNA interactions in solution.
- Diagnostic Assays: RNA EMSA-based assays are being developed for diagnosing RNA-related diseases and conditions, such as viral infections and cancer biomarker detection.
Components and Materials:
- RNA Samples:
- RNA molecules of interest, which can be synthesized in vitro or isolated from cells or tissues.
- Often labeled with radioactive or fluorescent tags for visualization.
- Proteins or Ligands:
- Proteins or ligands that interact with RNA molecules.
- Purified proteins for RNA-protein interaction studies or specific ligands for RNA-ligand interaction assays.
- Buffers:
- Various buffer solutions to create optimal conditions for RNA-protein or RNA-ligand binding.
- May include binding buffer, running buffer, and gel loading buffer.
- Gel Matrix:
- Typically a polyacrylamide gel with a defined concentration.
- The gel matrix retards the mobility of RNA-protein or RNA-ligand complexes during electrophoresis.
- Electrophoresis Apparatus:
- An electrophoresis chamber and power supply for running the gel.
- Comb-shaped well-formers for loading samples into the gel.
- Gel Visualization Equipment:
- Equipment for visualizing RNA bands on the gel, depending on the labeling method.
- For radioactive labeling, this includes X-ray film or a phosphorimager.
- For fluorescent labeling, a fluorescence imager is used.
- Molecular Weight Markers:
- Size standards or molecular weight markers used to estimate the size of RNA-protein or RNA-ligand complexes.
- Pipettes and Tips:
- To accurately measure and transfer reagents and samples during the assay.
- Cooling Equipment:
- Some experiments may require cooling equipment, such as a cold room or gel electrophoresis tank with temperature control.
- Safety Equipment:
- Appropriate personal protective equipment (PPE) and safety measures, especially when using radioactive materials or hazardous chemicals.
- Laboratory Supplies:
- General lab supplies like microcentrifuge tubes, pipette tips, and gloves for handling samples.
- Analytical Instruments:
- Instruments for quantifying RNA and protein concentrations, such as spectrophotometers or fluorometers.
Experimental Procedure:
1. Preparation of RNA and Proteins/Ligands:
- Synthesize or isolate the RNA molecules of interest.
- Label the RNA with a radioactive or fluorescent tag if necessary for detection.
- Purify the proteins or prepare the ligands that will interact with the RNA.
2. Preparation of Buffers:
- Prepare the necessary buffers, including binding buffer and running buffer, according to the specific requirements of your experiment.
3. RNA-Protein/Ligand Binding:
- Mix the labeled RNA with the purified proteins or ligands in a microcentrifuge tube.
- Incubate the mixture at an appropriate temperature and time to allow complex formation.
4. Preparation of Polyacrylamide Gel:
- Prepare a polyacrylamide gel with the desired concentration and dimensions.
- Cast the gel in a gel-casting tray, and insert comb-shaped well-formers for sample loading.
5. Loading Samples onto the Gel:
- Load the RNA-protein or RNA-ligand mixture and control samples (free RNA) into the wells of the gel using a micropipette.
- Include a molecular weight marker lane for reference.
6. Electrophoresis:
- Place the gel into an electrophoresis chamber filled with running buffer.
- Apply an electric field by connecting the chamber to a power supply.
- Run the gel at a low voltage to prevent the disruption of RNA-protein or RNA-ligand complexes.
- Monitor the progress of electrophoresis, typically for 1-2 hours or until the complexes have sufficiently separated from free RNA.
7. Gel Staining and Visualization:
- After electrophoresis, carefully remove the gel from the chamber.
- Stain the gel with a suitable dye (e.g., ethidium bromide for fluorescent detection) or expose it to X-ray film (for radioactive labeling).
- Visualize the RNA bands using an appropriate imaging system, such as a UV transilluminator or phosphorimager.
8. Data Analysis:
- Analyze the gel to determine the presence of shifted bands, indicating the formation of RNA-protein or RNA-ligand complexes.
- Measure the intensity of bands to assess binding affinity.
- Compare results with control lanes to confirm specificity.
9. Interpretation and Conclusion:
- Interpret the results to draw conclusions about the RNA interactions being studied.
- Summarize the findings and discuss their implications in the context of your research.
It’s important to note that the specific conditions and reagents used in each step may vary depending on the experiment’s requirements and objectives. Careful optimization of conditions is often necessary to achieve reliable results in RNA EMSA.
Interpreting EMSA Results:
- Band Patterns: Examine the gel for the presence of bands or shifts. There are typically three types of bands you might observe:
- Free Nucleic Acid Bands: These bands represent unbound nucleic acid molecules. They migrate the farthest and appear as the lowest bands on the gel.
- Shifted Bands: These bands represent nucleic acid molecules bound to proteins or ligands. They migrate more slowly than the free nucleic acid due to the added mass of the bound molecule(s). The number and intensity of shifted bands can vary depending on the binding affinity, stoichiometry, and specificity of the interaction.
- Super-shifted Bands (optional): In some cases, if you use antibodies or specific protein modifiers (e.g., specific antibodies or chemical crosslinkers), you might observe super-shifted bands, indicating that the protein-nucleic acid complex has been further altered or tagged.
- Control Lanes: Always include control lanes on your gel. These control lanes help you make sense of your results. Typically, control lanes consist of:
- Unbound Nucleic Acid Control: A lane with only the labeled nucleic acid (without the protein or ligand). This lane helps identify the position of free nucleic acid bands.
- Competition Assays (optional): By including lanes with excess unlabeled nucleic acid (competitor), you can assess the specificity of the interaction. If the competitor displaces the labeled nucleic acid from the complex, it indicates that the interaction is specific.
- Quantitative Analysis: Measure the intensity of the bands (free and shifted) using appropriate software or tools. The ratio of shifted band intensity to the total intensity (sum of shifted and free bands) can be used to estimate binding affinity.
- Binding Specificity: Consider whether the binding is specific. Specific binding will show shifted bands that are absent or reduced in the presence of excess unlabeled competitor or under specific conditions.
- Stoichiometry: Observe the number of shifted bands. Different band patterns can indicate the stoichiometry of the complex (e.g., one nucleic acid molecule bound to one protein vs. multiple molecules bound).
- Reproducibility: Ensure that your results are reproducible across multiple experiments. Variations in conditions, such as protein concentration, incubation time, or buffer composition, can affect the results.
Optimizing RNA EMSA:
- Design RNA Probes:
- Ensure that your RNA probes are well-designed with appropriate lengths and sequences.
- Consider using shorter probes if possible, as longer RNA molecules may form complex secondary structures that interfere with binding.
- RNA Labeling:
- Optimize labeling efficiency to ensure a sufficient signal-to-noise ratio.
- Choose the appropriate labeling method (e.g., radioactive or fluorescent) based on your equipment and detection capabilities.
- Purification of Proteins or Ligands:
- Purify your proteins or ligands to high homogeneity to minimize contaminants that could affect binding specificity.
- Verify the integrity and activity of your purified proteins using suitable assays.
- Buffer Optimization:
- Experiment with different binding buffers to find the optimal conditions for RNA-protein or RNA-ligand interactions.
- Adjust factors like salt concentration, pH, and additives to enhance binding.
- Incubation Conditions:
- Optimize the incubation temperature and time for complex formation. Some interactions may require longer incubation periods or specific temperatures.
- Use a gentle rocking or mixing to ensure thorough mixing of the reaction components.
- Gel Concentration and Running Conditions:
- Vary the polyacrylamide gel concentration to achieve better resolution. Lower percentages are better for larger complexes.
- Experiment with different gel running conditions, such as voltage and running time, to optimize the separation of free RNA from bound complexes.
- Loading Dye and Sample Preparation:
- Use appropriate loading dyes that do not interfere with binding. EDTA, for example, can chelate metal ions and affect protein binding.
- Ensure consistent sample loading by using consistent volumes and concentrations.
- Control Experiments:
- Include control lanes with free RNA only, RNA-protein or RNA-ligand complexes without competitors, and competitors (unlabeled RNA) to assess specificity.
- Use known binding partners or positive controls to validate your experimental setup.
- Reproducibility: Conduct replicate experiments to ensure consistency and reproducibility of results.
- Detecting Shifted Bands:
- Use appropriate detection methods for your labeled RNA (e.g., X-ray film for radioactive labels or fluorescence imaging for fluorescent labels).
- Optimize exposure times or imaging settings to capture bands clearly without oversaturation.
- Quantitative Analysis:
- Quantify band intensities using appropriate software to assess binding affinity.
- Perform dose-response experiments by varying protein or ligand concentrations to determine binding constants.
- Troubleshooting:
- If you encounter unexpected results or issues, troubleshoot systematically by adjusting one variable at a time to identify the problem source.
- Consult the Literature: Review published studies that have used similar RNA EMSA conditions for guidance and potential optimizations.
Advantages and Limitations:
Advantages:
- Specificity Assessment: RNA EMSA allows researchers to assess the specificity of RNA-protein or RNA-ligand interactions by competing with excess unlabeled RNA.
- Binding Affinity: It provides a means to estimate the binding affinity of interactions by measuring the intensity of shifted bands.
- Versatility: RNA EMSA is a versatile tool for studying RNA-protein and RNA-ligand interactions, applicable to various research areas.
- Insight into RNA Structure: It can offer insights into RNA secondary structure changes induced by binding partners.
- Comparative Studies: Researchers can compare different RNA sequences, mutants, or binding partners to dissect the molecular mechanisms of interactions.
Limitations:
- Sensitivity to Conditions: RNA EMSA results can be sensitive to experimental conditions, necessitating careful optimization.
- False Positives: It may yield false positives or nonspecific interactions under certain conditions, requiring validation with additional assays.
- Limited Quantification: Quantitative analysis of binding affinity is semi-quantitative and may not provide precise binding constants.
- Complexes Stability: The stability of RNA-protein or RNA-ligand complexes can vary, affecting the detectability of shifted bands.
- Time-Consuming: RNA EMSA can be time-consuming, especially when optimizing conditions or conducting dose-response experiments.
- Large RNAs: It is less effective for studying very large RNA molecules, as they may not migrate well in the gel.
- Radioactive Materials: If using radioactive labeling, safety concerns and disposal of radioactive waste can be a limitation.
Troubleshooting and Optimization:
Troubleshooting:
- Weak or No Shifted Bands:
- Issue: Insufficient binding or complex formation.
- Solution: Optimize conditions like protein concentration, incubation time, and buffer composition.
- High Background Noise:
- Issue: Non-specific binding or contamination.
- Solution: Use excess unlabeled RNA (competitor) to test for specificity and ensure thorough protein purification.
- Band Smearing:
- Issue: Formation of multiple complexes or instability.
- Solution: Experiment with different incubation times, temperatures, or stabilizing additives.
- No Signal from Labeled RNA:
- Issue: Poor labeling efficiency or degradation.
- Solution: Optimize labeling techniques and ensure RNA integrity.
- Inconsistent Replicates:
- Issue: Variability between replicate experiments.
- Solution: Standardize protocols, reagents, and equipment.
Optimization:
- Buffer Optimization:
- Goal: Adjust salt concentration, pH, and additives to enhance binding.
- Approach: Systematically vary buffer conditions while keeping other parameters constant.
- Incubation Conditions:
- Goal: Optimize incubation temperature and time for efficient complex formation.
- Approach: Test a range of temperatures and incubation durations.
- Protein Concentration:
- Goal: Determine the optimal protein concentration for maximal complex formation.
- Approach: Perform binding assays with varying protein concentrations.
- RNA Probe Design:
- Goal: Design RNA probes with appropriate lengths and sequences.
- Approach: Ensure probes are free from self-complementarity and secondary structures.
- Gel Concentration:
- Goal: Achieve better resolution of complexes.
- Approach: Experiment with different gel concentrations to find the most suitable one.
- Control Experiments:
- Goal: Verify the specificity and sensitivity of your assay.
- Approach: Include control lanes with known binding partners and competitors (unlabeled RNA).
- Reproducibility:
- Goal: Ensure consistent results between experiments.
- Approach: Conduct replicates and document procedures rigorously.
Advanced Techniques and Modifications:
- Supershift EMSA:
- Description: Involves using antibodies specific to the protein of interest to further characterize the RNA-protein complex.
- Application: Provides information about the identity of the protein in the complex.
- RNA EMSA with Mutant Proteins or RNAs:
- Description: Studying interactions using mutant proteins or RNAs to investigate the specific regions or motifs involved in binding.
- Application: Helps identify critical binding sites and functional domains.
- UV Crosslinking EMSA:
- Description: Utilizes UV irradiation to covalently link RNA-protein complexes, enhancing their stability for downstream analysis.
- Application: Enables additional experiments like RNA-protein co-immunoprecipitation (RIP) or mass spectrometry.
Modifications:
- Fluorescent EMSA (FEMSA):
- Description: Uses fluorescently labeled RNA for detection, offering improved sensitivity and reduced safety concerns compared to radioactive labels.
- Application: Suitable for real-time monitoring and high-throughput applications.
- Non-Radioactive Detection:
- Description: Replaces radioactive labeling with non-radioactive alternatives like chemiluminescence or biotin-streptavidin detection systems.
- Application: Enhances safety and simplifies disposal while maintaining sensitivity.
- Multiplex EMSA:
- Description: Allows simultaneous study of multiple RNA-protein interactions or RNA-ligand interactions within a single gel.
- Application: Efficiently screens for multiple interactions in parallel.
- RNA EMSA with Modified RNA Analogs:
- Description: Uses modified RNA analogs (e.g., 2′-O-methyl RNA) to investigate the impact of modifications on binding interactions.
- Application: Offers insights into the role of post-transcriptional RNA modifications in RNA-protein interactions.
Future Perspectives and Emerging Technologies:
- High-Throughput RNA EMSA: Develop automated systems and microfluidic platforms for high-throughput RNA EMSA, allowing simultaneous screening of numerous RNA-protein interactions or ligand binding events.
- Single-Molecule RNA EMSA: Adapt single-molecule techniques, such as single-molecule fluorescence microscopy or nanopore-based methods, to study RNA-protein interactions at the single-molecule level, providing detailed kinetics and heterogeneity information.
- Cryo-EM and Structural Insights: Combine RNA EMSA with cryo-electron microscopy (cryo-EM) to visualize RNA-protein complexes at high resolution, elucidating their structures and dynamic conformational changes.
- RNA-Sequencing Integration: Integrate RNA-seq data to identify RNA targets and potential binding partners, allowing comprehensive analysis of RNA-protein interactomes.
- Quantitative Mass Spectrometry: Implement quantitative mass spectrometry techniques to precisely measure binding stoichiometry and quantify changes in RNA-protein complexes.
- Super-Resolution Imaging: Apply super-resolution imaging techniques to visualize and track RNA-protein interactions in live cells with nanoscale precision.
- RNA Aptamers and SELEX: Develop RNA aptamers with high binding affinity and specificity for various proteins or ligands through systematic evolution of ligands by exponential enrichment (SELEX) methods.
- Functional RNA-Protein Studies: Expand RNA EMSA into functional assays that measure the impact of RNA-protein interactions on RNA processing, localization, translation, and degradation.
- High-Content Analysis: Combine RNA EMSA with high-content screening methods to assess the effects of small molecules, drugs, or genetic perturbations on RNA-protein interactions in a high-throughput manner.
- RNA-ligand Interactions: Investigate RNA-ligand interactions using RNA EMSA for drug discovery and therapeutic development, focusing on RNA-targeted therapies.
- Integration with CRISPR-Cas Systems: Combine RNA EMSA with CRISPR-Cas technologies to study the functional consequences of RNA-protein interactions on gene expression and regulation.
- Artificial Intelligence (AI) and Data Analysis: Implement AI-driven data analysis tools to automate band identification, quantify binding affinities, and predict binding motifs from EMSA results.
FAQs:
1. What is RNA EMSA, and what is its primary purpose?
- RNA EMSA (Electrophoretic Mobility Shift Assay) is a laboratory technique used to study RNA-protein or RNA-ligand interactions. Its primary purpose is to investigate the binding of RNA molecules to proteins or ligands and to assess binding specificity and affinity.
2. How does RNA EMSA work?
- RNA EMSA separates RNA-protein or RNA-ligand complexes from free RNA using gel electrophoresis. Complexes migrate more slowly through the gel, resulting in shifted bands, while free RNA moves faster.
3. What are the components needed for RNA EMSA?
- Components include RNA samples (labeled or unlabeled), proteins or ligands, buffers, a polyacrylamide gel, electrophoresis equipment, and detection methods (e.g., autoradiography or fluorescence).
4. How can I optimize RNA EMSA experiments?
- Optimization involves adjusting parameters like protein concentration, incubation conditions, buffer composition, and gel concentration. Systematic testing and adjustments are crucial for optimal results.
5. What are the advantages of using RNA EMSA?
- RNA EMSA offers specificity assessment, binding affinity estimation, versatility in studying various RNA interactions, insight into RNA secondary structure changes, and the ability to compare different RNA sequences or mutants.
6. What are the limitations of RNA EMSA?
- Limitations include sensitivity to experimental conditions, potential false positives, semi-quantitative binding affinity measurements, and sensitivity to RNA-protein complex stability.
7. What are some advanced techniques and modifications for RNA EMSA?
- Advanced techniques include supershift EMSA, RNA EMSA with mutant proteins or RNAs, UV crosslinking EMSA, fluorescent EMSA (FEMSA), non-radioactive detection, multiplex EMSA, and RNA EMSA with modified RNA analogs.
8. What emerging technologies are impacting RNA EMSA?
- Emerging technologies include high-throughput RNA EMSA, single-molecule RNA EMSA, cryo-EM integration, RNA sequencing integration, quantitative mass spectrometry, super-resolution imaging, and AI-driven data analysis.
9. How can I troubleshoot common issues in RNA EMSA experiments?
- Troubleshooting involves addressing problems like weak or no shifted bands, high background noise, band smearing, no signal from labeled RNA, and inconsistent replicates through systematic adjustments of experimental parameters.
10. What is the future outlook for RNA EMSA?
- The future of RNA EMSA involves integration with advanced technologies, functional studies, high-content analysis, and increased automation. It remains a valuable tool for studying RNA interactions and biology.
Conclusion:
In conclusion, RNA Electrophoretic Mobility Shift Assay (RNA EMSA) stands as a fundamental and versatile technique in the realm of molecular biology and biochemistry. This powerful assay enables researchers to explore the intricate interactions between RNA molecules and their binding partners, whether they be proteins, ligands, or other molecules.
RNA EMSA’s operational principles involve electrophoresis, with the separation of RNA-protein or RNA-ligand complexes from free RNA serving as the basis for its insightful results. Through the formation of shifted bands on a gel, researchers gain valuable information about binding specificity, affinity, and the structural dynamics of RNA.
Possible References Used