Gas Chromatography (GC) is a widely used analytical technique in chemistry and analytical chemistry for separating and analyzing volatile and semi-volatile compounds in a mixture. It is based on the principle of partitioning a sample between a stationary phase (usually a coated capillary column) and a mobile phase (an inert gas such as helium or nitrogen). The components in the sample are separated based on their different affinities for the stationary and mobile phases.
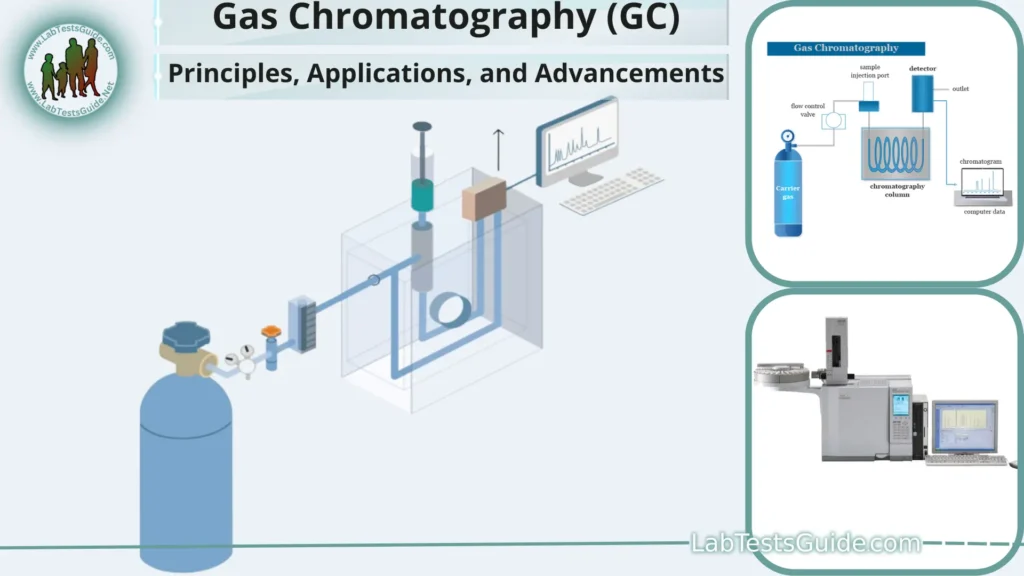
Gas Chromatography (GC) is a fundamental analytical technique that plays a pivotal role in modern scientific research and industry. With its remarkable ability to separate and quantify complex mixtures of volatile and semi-volatile compounds, GC has earned a prominent place in fields ranging from environmental analysis to pharmaceutical development. This comprehensive 3500-word article delves deep into the principles, applications, and recent advancements in Gas Chromatography.
Introduction
Definition and Historical Perspective
Gas Chromatography (GC) is a powerful analytical technique used to separate and analyze complex mixtures of volatile and semi-volatile compounds. It operates on the fundamental principles of selective partitioning and is invaluable in chemical analysis, scientific research, and various industrial applications.
The roots of GC can be traced back to the early 20th century when Mikhail Semenovich Tswett, a Russian-Italian botanist, first used a glass column packed with finely divided calcium carbonate to separate plant pigments. Tswett’s work laid the foundation for chromatography, a term derived from the Greek words “chroma” (color) and “graphein” (to write), reflecting its initial application in separating colored compounds.
Gas Chromatography as we know it today emerged in the 1950s with the development of modern instrumentation and stationary phases. Archer John Porter Martin and Richard Laurence Millington Synge, both British chemists, were awarded the Nobel Prize in Chemistry in 1952 for their groundbreaking work on liquid-liquid chromatography (LLC), which paved the way for the development of Gas Chromatography.
Importance in Analytical Chemistry
Gas Chromatography has since become an indispensable tool in analytical chemistry, offering exceptional precision, sensitivity, and versatility. It enables the separation and quantification of a wide range of compounds, including hydrocarbons, volatile organic compounds (VOCs), drugs, pesticides, and more. Its significance can be summarized as follows:
- Quantitative Analysis: GC provides accurate and reproducible quantitative data, making it a cornerstone of quality control and chemical analysis in various industries.
- Identification of Unknown Compounds: Through the retention times and spectra generated by detectors, GC aids in identifying unknown compounds and elucidating their structures.
- Complex Sample Analysis: It excels in separating complex mixtures, making it valuable in fields such as environmental analysis, food chemistry, and forensic science.
- Environmental Monitoring: GC is widely used for monitoring air and water quality, detecting pollutants, and studying volatile organic compounds (VOCs) involved in environmental processes.
- Drug Discovery and Development: Pharmaceutical companies rely on GC for the analysis of drug formulations, impurity profiling, and pharmacokinetic studies.
- Criminal Investigations: In forensic science, GC is pivotal for analyzing trace evidence, drugs of abuse, and volatile compounds associated with arson investigations.
As we delve deeper into Gas Chromatography, we’ll explore the fundamental principles that underlie its operation and the components that make up a typical GC system.
Principles of Gas Chromatography
Components of a GC System
A Gas Chromatograph is a complex analytical instrument comprised of several key components that work in tandem to separate and analyze compounds within a sample. The primary components include:
a. Inlet System:
- Sample Introduction: The sample is introduced into the GC system through an inlet system. Common techniques include split injection, splitless injection, and on-column injection.
b. Column:
- Stationary Phase: The column contains a stationary phase, which can be a solid or a liquid. The choice of stationary phase depends on the nature of the compounds being analyzed. Common stationary phases include silica, alumina, and various polymers.
- Column Length and Diameter: The column’s dimensions can vary, with longer columns providing better separation but requiring longer analysis times.
c. Oven:
- Temperature Control: The column is housed in an oven, which allows precise control of temperature. Temperature plays a crucial role in compound separation as it influences their vapor pressure and interaction with the stationary phase.
d. Detector:
- Compound Detection: Compounds exiting the column are detected by a specific detector. Different detectors are available, including the Flame Ionization Detector (FID), Thermal Conductivity Detector (TCD), Electron Capture Detector (ECD), and Mass Spectrometer (MS).
e. Data System:
- Data Acquisition and Analysis: A computerized data system records and analyzes the detector’s output, producing chromatograms that represent the compound separation and concentration.
Gas-Solid and Gas-Liquid Chromatography
Gas Chromatography can be categorized into two main types based on the interaction between the sample components and the stationary phase: Gas-Solid Chromatography (GSC) and Gas-Liquid Chromatography (GLC).
a. Gas-Solid Chromatography (GSC):
In GSC, the stationary phase is a solid adsorbent, and separation is achieved based on the adsorption and desorption of compounds on the solid surface. Common solid phases include activated charcoal, silica gel, and molecular sieves.
GSC is well-suited for the separation of gases and low molecular weight compounds. It is often used for the analysis of permanent gases, such as nitrogen, oxygen, and carbon dioxide, as well as simple hydrocarbons.
b. Gas-Liquid Chromatography (GLC):
In GLC, the stationary phase is a liquid coated on the surface of an inert solid support within the column. Separation occurs as compounds partition between the mobile gas phase and the liquid stationary phase.
GLC is highly versatile and is used for a wide range of compounds, including organic molecules, volatile and semi-volatile compounds, and polar substances. It is the most common form of Gas Chromatography.
Retention Time and Retention Factor
Key concepts in Gas Chromatography are retention time (tR) and retention factor (k or α). These parameters are central to compound identification and quantification.
a. Retention Time (tR):
Retention time (tR) is the time it takes for a compound to travel through the column from injection to detection. It is influenced by the compound’s interaction with the stationary phase, column temperature, and flow rate of the carrier gas.
Retention time is a characteristic property of a compound under specific chromatographic conditions. It is used for compound identification by comparing it to reference standards.
b. Retention Factor (k or α):
The retention factor (k) or separation factor (α) is a measure of how strongly a compound interacts with the stationary phase relative to the mobile phase. It is calculated using the following formula:
[k = \frac{(tR – t0)}{t0}]
Where:
- (tR) is the retention time of the compound.
- (t0) is the retention time of an unretained compound (a non-interacting compound, often the solvent or carrier gas).
A higher retention factor indicates stronger interaction with the stationary phase, resulting in longer retention times and better separation from other compounds.
Resolution and Selectivity
The effectiveness of a Gas Chromatography separation is often assessed using two critical parameters: resolution (R) and selectivity (α).
a. Resolution (R):
Resolution is a measure of the separation between two adjacent peaks in a chromatogram. It quantifies how well two compounds are separated and is calculated using the following formula:
[R = \frac{2\Delta tR}{W1 + W2}]
Where:
- (\Delta tR) is the difference in retention times between the two compounds.
- (W1) is the peak width of the first compound.
- (W2) is the peak width of the second compound.
High resolution is desirable as it allows for the accurate quantification and identification of compounds in a mixture.
b. Selectivity (α):
Selectivity (α) measures the ability of the column to separate two compounds. It is the ratio of their retention factors (k) and is calculated as follows:
[α = \frac{k2}{k1}]
Where:
- (k1) is the retention factor of the first compound.
- (k2) is the retention factor of the second compound.
A selectivity value greater than 1 indicates good separation between the two compounds, while a value less than 1 suggests poor separation.
These fundamental principles govern the operation of Gas Chromatography and form the basis for optimizing separation conditions and interpreting chromatographic results. In the next section, we explore the essential components of a Gas Chromatograph in greater detail.
Instrumentation
A Gas Chromatograph consists of several critical components that work together to separate and analyze compounds in a sample. Understanding the instrumentation is essential for operating the GC effectively and optimizing analytical results.
Gas Supply and Flow Control
The carrier gas used in Gas Chromatography serves two primary functions: it transports the sample through the column and acts as a mobile phase. Helium and nitrogen are commonly used carrier gases due to their inertness and ease of handling. Hydrogen is occasionally used but requires special precautions due to its flammable nature.
a. Gas Cylinder:
Gas Chromatographs are connected to gas cylinders containing the carrier gas. The gas flow rate is controlled using a flow regulator, which allows precise adjustment of the carrier gas flow.
b. Split/Splitless Injector:
The injector is the point at which the sample is introduced into the GC system. There are two primary injection techniques:
- Split Injection: In split injection, only a portion of the sample introduced into the inlet goes into the column. The rest is vented out, reducing the amount of sample that enters the column. This technique is useful for preventing column overload with concentrated samples.
- Splitless Injection: In splitless injection, the entire sample is introduced into the column. It is particularly useful for trace analysis when maximum sensitivity is required.
Sample Introduction Systems
Sample introduction is a critical step in Gas Chromatography, as it can impact the quality of the chromatographic separation and detection. Various sample introduction techniques are used based on the nature of the sample.
a. Liquid Sample Introduction:
- Syringe Injection: For liquid samples, a microsyringe is used to inject a precise volume of the sample into the injector port. This technique is commonly used in GLC.
- On-Column Injection: In on-column injection, the sample is introduced directly onto the head of the GC column. It is often used for samples that may undergo degradation or adsorption in the injector.
b. Gas Sample Introduction:
- Gas Sample Loop: For gaseous samples, a gas sample loop is used to introduce a known volume of the sample into the carrier gas stream. This technique is commonly used in GSC.
GC Columns
The column is a critical component of a Gas Chromatograph, and its properties can significantly impact separation. Columns come in various dimensions, materials, and stationary phases to accommodate different applications.
a. Column Length and Diameter:
- Column Length: Longer columns provide better separation but require longer analysis times. Short columns are suitable for rapid analyses.
- Column Diameter: The column’s inner diameter can vary, with smaller diameters offering higher resolution but potentially longer analysis times.
b. Stationary Phase:
- Liquid Stationary Phase: Most GC columns use a liquid stationary phase coated onto an inert solid support. The choice of stationary phase depends on the types of compounds being analyzed. Common phases include:
- Polar Phases: Used for separating polar compounds (e.g., polar capillary columns with polyethylene glycol stationary phases).
- Nonpolar Phases: Suitable for nonpolar compounds (e.g., dimethylpolysiloxane phases).
- Specialty Phases: Some phases are designed for specific applications, such as separating pesticides or volatile organic compounds (VOCs).
- Solid Stationary Phase: In Gas-Solid Chromatography, the stationary phase is a solid adsorbent, typically activated charcoal or molecular sieves.
Detectors in Gas Chromatography
The detector is a critical component of a Gas Chromatograph, as it is responsible for converting the eluting compounds into measurable signals. Different detectors are used based on the specific analytical requirements.
a. Flame Ionization Detector (FID):
The Flame Ionization Detector (FID) is one of the most widely used detectors in GC. It operates based on the combustion of organic compounds in a hydrogen-air flame. Key features of the FID include:
- High sensitivity and wide linear range.
- Suitable for detecting hydrocarbons and most organic compounds.
- Non-destructive, allowing for sample collection after detection.
- Produces a response proportional to the carbon content of the compounds.
b. Thermal Conductivity Detector (TCD):
The Thermal Conductivity Detector (TCD) measures changes in the thermal conductivity of the carrier gas caused by the presence of analyte molecules. Key features include:
- Universal detection for all compounds, regardless of their chemical properties.
- Suitable for analyzing permanent gases.
- Limited sensitivity compared to other detectors.
c. Electron Capture Detector (ECD):
The Electron Capture Detector (ECD) relies on the capture of electrons by electronegative atoms or molecules in the sample. Key features include:
- High sensitivity for compounds containing electronegative elements (e.g., halogens).
- Selective for compounds with electron-capturing capabilities.
- Requires a radioactive source (typically nickel-63) to generate electrons.
d. Mass Spectrometry (MS):
Mass Spectrometry (MS) is a highly selective and versatile detection technique that can provide compound identification based on mass-to-charge ratios. Key features include:
- Exceptional sensitivity and specificity.
- Provides molecular weight and structural information.
- Allows for the identification of unknown compounds.
- Often coupled with GC to form Gas Chromatography-Mass Spectrometry (GC-MS) systems.
Each detector has its advantages and limitations, and the choice of detector depends on the type of compounds being analyzed and the analytical goals. GC-MS, in particular, is a powerful combination that offers both separation and compound identification capabilities.
In the following section, we explore sample preparation techniques and the role of derivatization in Gas Chromatography.
Sample Preparation and Derivatization
Sample preparation is a crucial step in Gas Chromatography, especially when dealing with complex or non-volatile samples. It involves various techniques to ensure that the sample is compatible with the chromatographic system and can yield accurate and reproducible results.
Sample Types and Preparation Techniques
Samples for Gas Chromatography analysis can be broadly categorized into liquid, solid, or gaseous forms. The choice of sample preparation technique depends on the sample’s nature and the analytical goals.
a. Liquid Samples:
- Direct Injection: For relatively clean liquid samples, direct injection using a syringe is a common technique. It involves injecting a small volume of the liquid sample into the GC inlet.
- Liquid-Liquid Extraction: When the sample contains multiple components or interferents, liquid-liquid extraction may be necessary to isolate the analyte. This technique involves mixing the sample with an organic solvent that selectively extracts the analyte, followed by injection of the solvent into the GC.
- Solid-Phase Microextraction (SPME): SPME is a solvent-free technique where a coated fiber absorbs volatile and semi-volatile compounds from the sample matrix. The fiber is then introduced into the GC inlet for thermal desorption.
b. Solid Samples:
- Headspace Sampling: This technique is used for solid or liquid samples that contain volatile compounds. It involves heating the sample in a sealed vial, allowing the volatile compounds to equilibrate in the headspace above the sample, and then injecting the headspace vapor into the GC.
- Extraction: Solid samples can be subjected to various extraction techniques, such as solid-phase extraction (SPE) or Soxhlet extraction, to isolate analytes. The resulting extract is then injected into the GC.
c. Gaseous Samples:
- Gas Sampling: Gaseous samples can be directly introduced into the GC using gas sampling valves or gas loops.
Derivatization in GC
Derivatization is a chemical technique used to modify the chemical structure of analytes to improve their volatility, thermal stability, or detectability by specific detectors. It is particularly useful for compounds that are not inherently amenable to Gas Chromatography analysis. Common objectives of derivatization include:
- Increased Volatility: Some analytes are non-volatile or have low volatility. Derivatization can convert them into more volatile derivatives, making them suitable for GC analysis.
- Enhanced Thermal Stability: Certain compounds may decompose or degrade at high temperatures in the GC column. Derivatization can increase their thermal stability.
- Improved Detection: Derivatization can introduce functional groups or chemical properties that enhance the compound’s detectability with specific detectors, such as the FID or ECD.
- Better Separation: Derivatization can change a compound’s chemical structure, affecting its interaction with the stationary phase and leading to improved separation.
Common derivatization reactions include silylation, acylation, alkylation, and esterification. For example, the silylation of alcohols or acids with trimethylsilyl (TMS) reagents can increase their volatility and improve their chromatographic behavior.
The choice of derivatization reagent and reaction conditions depends on the analyte’s chemical structure and the desired analytical outcomes. Derivatization should be carefully optimized to ensure that it does not introduce artifacts or interfere with compound quantification.
In the following sections, we delve into various Gas Chromatography techniques, including temperature programming, Gas Chromatography-Mass Spectrometry (GC-MS), and recent advancements in the field.
Gas Chromatography Techniques
Gas Chromatography offers several techniques and strategies to optimize compound separation, enhance sensitivity, and achieve specific analytical goals. Understanding these techniques is essential for tailoring GC methods to different applications.
Temperature Programming
Temperature programming is a common technique used to improve the separation efficiency and speed of GC analyses. It involves changing the column temperature during the analysis to exploit differences in compound volatility and interactions with the stationary phase.
a. Isothermal Analysis:
In isothermal analysis, the column temperature is held constant throughout the analysis. This approach is suitable for samples with a narrow boiling point range and when rapid analysis is required.
b. Temperature Ramp or Gradient:
Temperature programming involves gradually increasing the column temperature during the analysis. Key benefits include:
- Improved separation of compounds with a wide range of boiling points.
- Enhanced resolution and reduced analysis time.
- Better control over the elution of early and late-eluting compounds.
The temperature program is carefully designed based on the specific sample and separation objectives.
Gas Chromatography-Mass Spectrometry (GC-MS)
Gas Chromatography-Mass Spectrometry (GC-MS) is a powerful hyphenated technique that combines the separation capabilities of GC with the compound identification capabilities of Mass Spectrometry (MS).
a. Separation by GC:
In a GC-MS system, the GC component first separates the sample into its individual components as discussed earlier. This separation is based on the compounds’ retention times and interactions with the column.
b. Mass Spectrometry Detection:
After separation, the eluting compounds are directed into the mass spectrometer. In the MS component, compounds are ionized, fragmented, and their mass-to-charge ratios (m/z) are measured. This generates mass spectra that provide information about the compounds’ molecular structures.
c. Compound Identification:
The mass spectra obtained can be compared to reference libraries, allowing for the identification of compounds based on their unique mass fragmentation patterns. GC-MS is particularly valuable for identifying unknown compounds and is widely used in environmental analysis, forensic science, and the pharmaceutical industry.
GC-MS systems are versatile and can be configured with various ionization techniques, including electron impact (EI) and chemical ionization (CI), depending on the sample type and analytical requirements.
Two-Dimensional Gas Chromatography (2D-GC)
Two-Dimensional Gas
Chromatography (2D-GC or GCxGC) is an advanced chromatographic technique that significantly enhances separation capabilities. It involves coupling two GC columns with different stationary phases to achieve superior resolution.
a. First-Dimension Column:
The first-dimension column is similar to a standard GC column and is used for the initial separation of the sample components.
b. Modulator:
A modulator is placed between the first- and second-dimension columns. It collects and refocuses the compounds eluting from the first column before transferring them to the second column.
c. Second-Dimension Column:
The second-dimension column has a different stationary phase compared to the first column. It further separates the compounds based on their chemical properties.
2D-GC provides several advantages:
- Enhanced peak capacity and separation efficiency.
- Improved resolution of co-eluting compounds.
- Enhanced peak identification and quantification.
- Increased sensitivity.
This technique is particularly useful for analyzing complex mixtures where traditional GC may result in overlapping peaks.
High-Resolution Gas Chromatography (HRGC)
High-Resolution Gas Chromatography (HRGC) systems employ specialized columns and detectors to achieve exceptional separation capabilities. HRGC is especially valuable when analyzing complex mixtures or trace-level components.
a. Narrow-Bore Columns:
HRGC often uses narrow-bore columns with smaller inner diameters. These columns provide higher efficiency and better resolution but may require specialized instrumentation.
b. Specialty Detectors:
HRGC may utilize highly sensitive detectors, such as the Atomic Emission Detector (AED) or the Pulsed Flame Photometric Detector (PFPD). These detectors offer superior detection limits and specificity.
c. Comprehensive Separation:
HRGC aims to achieve comprehensive separation of all sample components, allowing for the detection and quantification of even trace-level compounds.
HRGC is commonly used in applications such as environmental analysis, food safety, and the characterization of complex natural product mixtures.
In the following section, we explore various applications of Gas Chromatography across different industries and fields of science.
Applications of Gas Chromatography
Gas Chromatography finds extensive use in various industries and scientific disciplines due to its ability to separate and quantify complex mixtures of compounds. Below are some notable applications of GC:
Environmental Analysis
Environmental monitoring and analysis are critical for assessing air and water quality, detecting pollutants, and understanding the impact of human activities on ecosystems. Gas Chromatography plays a vital role in environmental science by enabling the detection and quantification of various environmental contaminants, including:
- Volatile Organic Compounds (VOCs): GC is used to analyze VOCs emitted from industrial processes, transportation, and natural sources. Monitoring VOCs is essential for air quality assessment and identifying pollution sources.
- Pesticides and Herbicides: GC is employed to determine pesticide residues in soil, water, and food samples, ensuring compliance with safety regulations.
- Polychlorinated Biphenyls (PCBs) and Polycyclic Aromatic Hydrocarbons (PAHs): These persistent organic pollutants can be analyzed by GC to assess their presence in the environment and their potential health risks.
- Chlorinated Compounds: GC is used to monitor chlorinated solvents and other chlorine-containing compounds in groundwater and soil.
Food and Beverage Industry
The food and beverage industry relies on GC for quality control, flavor analysis, and safety testing. Some applications include:
- Flavor and Aroma Analysis: GC is used to identify and quantify volatile compounds responsible for the flavors and aromas of food and beverages.
- Pesticide Residue Analysis: GC helps detect and quantify pesticide residues in agricultural products to ensure food safety.
- Alcohol and Volatile Compound Analysis: GC is used in the brewing and distillation industries to monitor the production of alcoholic beverages and analyze volatile compounds.
- Fatty Acid Profiling: GC is employed to analyze fatty acids in oils and fats, helping to assess nutritional quality and shelf life.
Pharmaceutical Analysis
Pharmaceutical companies use Gas Chromatography for drug development, quality control, and regulatory compliance. GC applications in the pharmaceutical industry include:
- Drug Formulation Analysis: GC is used to analyze drug formulations, ensuring the presence of active ingredients and assessing impurities.
- Pharmacokinetic Studies: GC is applied to the analysis of drug metabolites and their concentrations in biological samples.
- Residual Solvent Analysis: GC is used to detect and quantify residual solvents in pharmaceutical products to ensure compliance with safety regulations.
- Stability Testing: GC helps assess the stability of pharmaceutical formulations over time, ensuring product quality and efficacy.
Petrochemical Industry
The petrochemical industry relies heavily on Gas Chromatography for quality control, process optimization, and product characterization. Key applications include:
- Hydrocarbon Analysis: GC is used to analyze hydrocarbon fractions in crude oil, refined products, and natural gas.
- Characterization of Petroleum Products: GC helps identify and quantify various components in fuels, lubricants, and petrochemical feedstocks.
- Sulfur Compound Analysis: GC is essential for monitoring sulfur compounds in fuels, as excessive sulfur levels can lead to environmental and operational issues.
- Trace Contaminant Analysis: GC is used to detect trace impurities and contaminants in petrochemical products, ensuring product quality and compliance with regulatory limits.
Forensic Science
Gas Chromatography plays a crucial role in forensic science by assisting in the analysis of evidence related to criminal investigations. Applications include:
- Drugs of Abuse Testing: GC is used to analyze drugs of abuse in biological samples, such as urine and blood, aiding in drug-related investigations.
- Arson Investigations: GC helps identify accelerants and volatile residues in fire debris, providing evidence in arson cases.
- Toxicology Analysis: GC is employed to analyze toxic compounds and poisons in post-mortem samples.
- Analysis of Trace Evidence: GC is used to analyze volatile compounds associated with trace evidence, such as fibers and gunshot residues.
Clinical Diagnostics
In clinical laboratories, Gas Chromatography is used for diagnostic purposes and the analysis of biological samples. Applications include:
- Blood Alcohol Analysis: GC is used to measure blood alcohol levels in forensic and clinical settings, such as DUI cases and emergency medicine.
- Hormone Analysis: GC is applied to analyze hormones and steroids in blood and urine samples for diagnostic purposes.
- Volatile Organic Compound Analysis: GC can detect volatile compounds associated with certain medical conditions, such as breath analysis for diabetes management.
- Pharmaceutical Analysis: GC is used to analyze pharmaceutical compounds and drug metabolites in biological samples during drug development and clinical trials.
These diverse applications highlight the versatility and importance of Gas Chromatography in various fields. As technology advances, GC continues to find new applications and contribute to scientific discoveries and industrial advancements.
In the following section, we explore recent advancements and innovations in Gas Chromatography, including miniaturization, comprehensive two-dimensional GC (GCxGC), hyphenated techniques, and the integration of artificial intelligence.
Advancements in Gas Chromatography
The field of Gas Chromatography has seen significant advancements in recent years, driven by innovations in instrumentation, technology, and analytical methods. These advancements have expanded the capabilities of GC and improved its efficiency and sensitivity. Here are some notable developments:
Miniaturization and Portable GC
Miniaturization of Gas Chromatography systems has led to the development of portable
and field-deployable GC instruments. These compact devices offer advantages such as:
- Portability: Portable GC systems are lightweight and compact, making them suitable for on-site analysis in remote locations or field applications.
- Rapid Analysis: Miniaturized GC systems often feature faster analysis times, enabling real-time or near-real-time results.
- Reduced Sample Volume: These systems require smaller sample volumes, making them ideal for applications where sample quantity is limited.
Portable GC systems have found applications in environmental monitoring, food safety inspections, and the detection of volatile organic compounds (VOCs) in various settings, including industrial workplaces and disaster response scenarios.
Comprehensive Two-Dimensional Gas Chromatography (GCxGC)
Comprehensive Two-Dimensional Gas Chromatography (GCxGC) has become a powerful technique for enhancing the separation capabilities of Gas Chromatography. In GCxGC:
- Two Columns: Two GC columns with different stationary phases are used in series.
- Modulation: A modulator collects and transfers analytes from the first column to the second column in a controlled manner.
- Enhanced Separation: GCxGC provides superior peak capacity, significantly improving the separation of complex mixtures.
GCxGC is particularly effective in applications where traditional GC struggles with peak overlap or where a more comprehensive analysis is needed, such as in petrochemical analysis, environmental monitoring, and food aroma profiling.
Hyphenated Techniques
Hyphenated techniques combine Gas Chromatography with other analytical methods to provide complementary information and enhance compound identification and quantification. Common hyphenated techniques include:
- Gas Chromatography-Mass Spectrometry (GC-MS): GC-MS combines the separation capabilities of GC with the compound identification capabilities of Mass Spectrometry. It is widely used for compound identification and quantification in diverse applications.
- Gas Chromatography-Infrared Spectroscopy (GC-IR): GC-IR combines GC with Infrared Spectroscopy to provide information about a compound’s molecular structure based on its IR spectrum. It is valuable for characterizing functional groups.
- Gas Chromatography-Flame Ionization Detection (GC-FID): GC-FID combines GC with Flame Ionization Detection, allowing for the quantification of compounds based on their carbon content. It is commonly used in petrochemical analysis.
- Gas Chromatography-Electron Capture Detection (GC-ECD): GC-ECD combines GC with Electron Capture Detection, making it highly sensitive to compounds containing electronegative elements like halogens and nitro groups.
Hyphenated techniques enhance the analytical capabilities of Gas Chromatography and provide valuable information about compound structure and properties.
Artificial Intelligence in GC Analysis
Artificial intelligence (AI) and machine learning (ML) techniques are increasingly being integrated into Gas Chromatography data analysis. AI-driven GC analysis offers several advantages:
- Automated Peak Identification: AI algorithms can automatically identify and quantify peaks in chromatograms, reducing the need for manual data interpretation.
- Pattern Recognition: ML algorithms can recognize complex patterns in chromatographic data, aiding in compound identification and sample classification.
- Predictive Modeling: AI can be used to develop predictive models for compound behavior, retention times, and peak shapes.
By harnessing AI and ML, researchers and analysts can streamline GC data analysis, reduce errors, and gain deeper insights from complex chromatographic data sets.
These advancements in Gas Chromatography continue to expand its applications, improve analytical capabilities, and make GC more accessible for a broader range of users. As technology evolves, GC will likely remain at the forefront of analytical chemistry, enabling new discoveries and innovations.
Challenges and Limitations
While Gas Chromatography offers numerous advantages, it is not without its challenges and limitations. Analysts and researchers should be aware of these factors to ensure accurate and reliable results.
Sample Matrix Effects
Sample matrices, such as complex mixtures or matrices with high levels of interfering compounds, can pose challenges in Gas Chromatography. These effects can lead to:
- Peak Overlapping: Complex matrices can cause peak overlap, making it difficult to separate and quantify individual compounds.
- Suppression or Enhancement: Matrix effects can suppress or enhance the response of analytes in the detector, leading to inaccurate quantification.
- Column Contamination: The presence of matrix components can contaminate the GC column, reducing column lifetime and performance.
To mitigate matrix effects, analysts may employ sample preparation techniques, such as sample cleanup, extraction, and derivatization, to simplify the sample matrix and improve analytical accuracy.
Non-Volatile Compounds
Gas Chromatography is primarily suited for the analysis of volatile and semi-volatile compounds. Non-volatile compounds, such as high-molecular-weight polymers and some metals, are not amenable to GC analysis due to their low volatility.
For the analysis of non-volatile compounds, other analytical techniques, such as Liquid Chromatography (LC) or Inductively Coupled Plasma-Mass Spectrometry (ICP-MS), may be more appropriate.
Column Overloading
Column overloading occurs when the amount of sample injected exceeds the column’s capacity to separate and resolve the compounds. This can result in:
- Peak Broadening: Overloading leads to broader peaks and decreased resolution.
- Loss of Linearity: Calibration curves may become nonlinear, affecting quantification accuracy.
- Column Damage: Overloading can lead to irreversible damage to the GC column.
To avoid column overloading, analysts should carefully select the appropriate injection volume and, if necessary, dilute the sample to ensure that peak separation is maintained.
Despite these challenges and limitations, Gas Chromatography remains a versatile and indispensable analytical technique. With proper method development, optimization, and sample preparation, many of these challenges can be effectively addressed.
Future Trends and Prospects
The future of Gas Chromatography holds exciting possibilities and trends that are likely to shape the field in the coming years. Some key trends and prospects include:
Enhanced Sensitivity and Selectivity
Continued advancements in GC instrumentation and column technology are expected to lead to improved sensitivity and selectivity. This will enable the detection and quantification of compounds at lower concentrations, making GC applicable to an even wider range of applications.
Green and Sustainable GC
Environmental concerns and sustainability efforts are driving the development of green analytical techniques. Future GC systems are likely to focus on reducing energy consumption, using eco-friendly solvents, and minimizing waste generation while maintaining high analytical performance.
Automation and Integration
Automation and integration of sample preparation, injection, analysis, and data processing are expected to become more prevalent. Automated GC systems will offer increased efficiency, reproducibility, and reduced operator workload.
Emerging Applications
As new analytical challenges arise in various industries, Gas Chromatography will continue to find applications in emerging fields. These may include the
analysis of advanced materials, biotechnology products, and nanomaterials, among others.
Integration with Omics Technologies
Integrating GC with omics technologies, such as metabolomics and proteomics, will provide a more comprehensive understanding of complex biological systems. This integration will aid in biomarker discovery, disease diagnostics, and drug development.
Artificial Intelligence and Big Data
The integration of artificial intelligence (AI) and big data analytics will play a significant role in GC data interpretation. Machine learning algorithms will assist in pattern recognition, compound identification, and predictive modeling, making data analysis faster and more accurate.
Miniaturization and Point-of-Care Testing
Miniaturized GC systems and point-of-care testing devices will become more accessible and play a crucial role in healthcare, environmental monitoring, and food safety applications.
Customized GC Systems
Customized GC systems tailored to specific applications and industries will become more prevalent. These systems will offer specialized solutions for unique analytical challenges.
The future of Gas Chromatography is bright, with continued innovation and adaptation to meet the evolving needs of science and industry. Researchers and analysts in various fields will continue to rely on GC as a powerful analytical tool for compound separation, identification, and quantification.
Conclusion
Gas Chromatography is a versatile and widely used analytical technique that plays a pivotal role in diverse scientific disciplines and industries. Its ability to separate, identify, and quantify compounds in complex mixtures has made it an indispensable tool for analytical chemists, researchers, and quality control professionals.
This comprehensive article has explored the fundamental principles of Gas Chromatography, its essential components, sample preparation techniques, and various GC techniques, including temperature programming, GC-MS, GCxGC, and HRGC. We have also discussed applications across environmental analysis, the food and beverage industry, pharmaceuticals, the petrochemical sector, forensic science, clinical diagnostics, and more.
Furthermore, we’ve highlighted recent advancements in Gas Chromatography, including miniaturization, hyphenated techniques, AI integration, and the future trends that are shaping the field.
While Gas Chromatography has its challenges and limitations, its continued evolution promises enhanced analytical capabilities, greater sustainability, and broader applications. As technology progresses and analytical needs evolve, Gas Chromatography will remain at the forefront of scientific research and industrial processes, contributing to new discoveries and innovations for years to come.
Possible References Used
One Comment